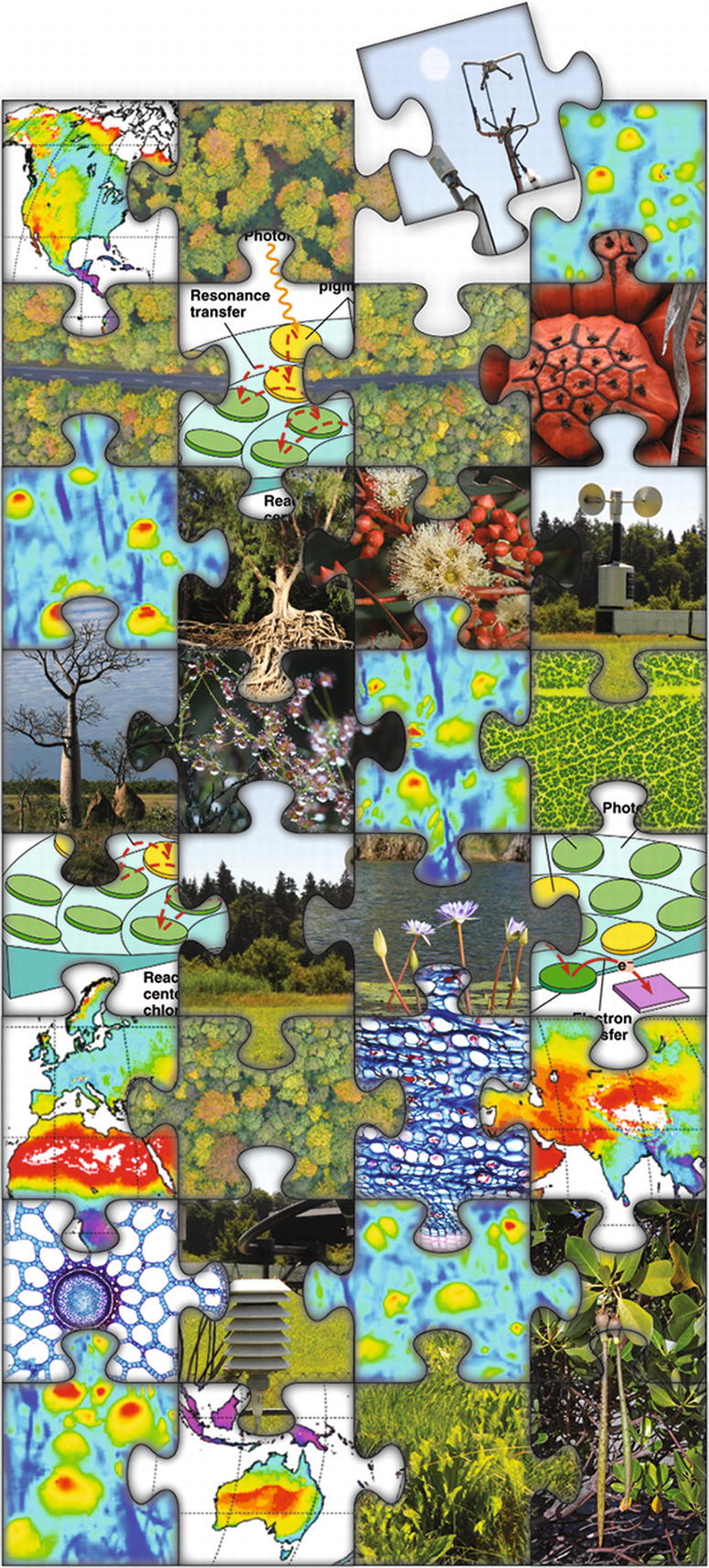
The puzzle illustrates the characteristics of modern Plant Ecology which aims to relate genetic information and molecular physiology with the biological and environmental constraints of plant life across the globe. Many parts of this puzzle are still not well known nor understood. This new edition of “Plant Ecology” aims to bridge these gaps, linking molecular stress physiology with plant performance, identifying processes which drive plant coexistence in plant communities, and assessing interactions of terrestrial ecosystems with their environment under past, current and future conditions.
The term “ecology” was defined by Ernst Haeckel in 1866 in his book Generelle Morphologie der Organismen [General Morphology of Organisms], as follows: “Ecology is the science of relations of the organism to the surrounding environment which includes, in its broadest sense, all ‘conditions for existence’. These conditions may be organic or inorganic; both are of the greatest importance for the form of organisms, because they force the organism to adjust.”
Haeckel included in the science of ecology the research fields of physiology, morphology, phylogeny and chorology (the science of the distribution of organisms) to understand the “conditions for existence” and the “adjustment of organisms”. Today we define the conditions for existence in a very broad sense, taking abiotic and also biotic interactions into account, even among different organism groups such as plants, animals and microorganisms. Haeckel’s term “adjustment” is today differentiated into “acclimation” and “adaptation”. While acclimation describes reversible and rather short-term adjustments of the physiology or morphology of plant individuals to the environment or biotic interactions, adaptation describes genetic adjustments mainly of populations over longer time scales.
Plants are sessile. Thus, they cannot physically escape predators and spreading pathogens, search for pollinators or hide from approaching extreme climatic situations. For example, forest understorey herbs rest in the deep shade of the tree canopy but are suddenly hit by an intense beam of direct sunlight as sunflecks move across the forest floor. To avoid damage to the shade-adapted light-harvesting machinery from high light intensity, leaves can change their orientation in space. Chloroplasts can move to the edges of a cell, and the antennae of light-harvesting complex II can be redistributed between photosystems II and I. Obviously, plants, as sessile organisms, must continuously cope with changing environmental conditions—from minute-by-minute to daily, seasonal and decadal fluctuations in temperature and light; changes in nutrient and water availability; and herbivore pressure, pathogen load and loss of mutualistic partners. Plants have therefore evolved a variety of mechanisms that enables them to tolerate and withstand environmental change, and to re-achieve internal homeostasis: plants are highly plastic and resilient to cope with a highly dynamic environment. Moreover, plants shape their own environment when growing together in a plant community, creating a three-dimensional canopy that affects microclimatic variables impacting on plant traits and plant performance. This microclimate, together with litter chemistry and litter production, controls water and biogeochemical fluxes on the ecosystem scale, adding to landscape heterogeneity and connectivity.
All of these aspects are addressed in this revision of the book Plant Ecology, expanding the very original views of ecology put forward by Haeckel (1866), Tansley (1935) and many others since then. Starting with molecular stress physiology and then focusing on physiological and biophysical plant ecology and ecosystem ecology, we place plant communities and terrestrial ecosystems in a global context. Thus, this book integrates different perspectives on plant ecology and presents these perspectives in five major parts, each divided into several chapters. In Part I, we start with Molecular Stress Physiology, which builds the basis for plant existence in a varying environment. We continue in Part II with the Physiological and Biophysical Plant Ecology. In Part III we focus on Ecosystem Ecology, in Part IV on Community Ecology and in Part V on Global Ecology. Many links across these five parts facilitate reading and, hopefully, the understanding of plant ecological concepts and mechanisms on different organisational scales.
The molecular mechanisms of stress responses as the basis for “adjustments” are outlined in Chap. 2. On the basis of this molecular foundation, the responses to the main environmental stresses are discussed. The model plant is often Arabidopsis thaliana, for which the total genome is known—an excellent prerequisite for linking genetic information to ecology. Thus, Part I of this book focuses on the effects of light (Chap. 3), temperature (Chap. 4), oxygen (Chap. 5), water (Chap. 6) and salt and soil factors (Chap. 7). The molecular level of biological interactions (Chap. 8) is explained in terms of signalling and stress responses.
At the level of the plant organism, we consider the responses of plant individuals to their environment, including plant structure, eco-physiological change in gas exchange and nutrient relations to the life cycles of various plant life forms (phenology, lifespan and strategies for reproduction and distribution). Although the active life of plants is typically limited to favourable conditions, there are mechanisms in long-lived plants that enable survival in unfavourable periods such as drought, heatwaves or frost. The scope for “adjustments” of plant organisms is very broad, like the responses of cells to stress. Plant responses range from changes in leaf structure to the differentiation of roots, and they include acclimation of biophysical processes during the exchange of substances with the environment. Thus, in Part II of this book the focus is on the use of resources by plant organisms. This includes their thermal balance (Chap. 9), water relations (Chap. 10), nutrient relations (Chap. 11) and carbon relations (Chap. 12).
Plants live in a neighbourhood with others and have to share resources with each other. Thus, water, nutrient and carbon relations are embedded in an ecosystem, where plants, animals and soil organisms interact with each other and their environment. This leads to questions as to whether ecosystems are more than the sum of their individual components and what emergent properties develop at this scale of organisation. Many characteristics of vegetation result in ecosystem-level matter fluxes, which control the performance of individual plants in this system. For example, the spatial structure of an ecosystem determines its coupling to the atmosphere, which may determine the survival of plant individuals or a species in this ecosystem. Part III of this book therefore introduces ecosystem characteristics and relevant concepts on the ecosystem scale (Chap. 13), and explains approaches to the study of terrestrial ecosystems (Chap. 14). One powerful approach to analysis of processes in complex systems is modelling (Chap. 15). Furthermore, we provide detailed insights into biogeochemical fluxes in terrestrial ecosystems (Chap. 16), since these processes are both drivers of, but also driven by global change.
Ecosystem processes and the spatial and temporal patterns of plant life are the basis for community ecology. Competition results from the limited amounts of resources that all plants rely on, growing adjacent to each other in limited space. If resources become scarce, efficiency means better use of limited resources at the cost of a neighbour. Of course, this does not always imply saving resources or using them most economically. Indeed, it may be more advantageous to use more resources than required, to pre-empt resources that will thus not be anymore available to competing neighbours. In community ecology, we learn that systems are very dynamic in the long-term and not all processes in a system change linearly in one direction. Community ecology does not consider the fate of a single individual plant or organ; it considers the dynamic spatial and temporal behaviour of populations, including growth and mortality. Generally, natural vegetation includes a diversity of species, which determine—in varying proportions—the composition of the vegetation cover of the Earth. Species differ in their characteristics, life strategies and traits, which may result in complementary use of available resources. Thus, the broad spectrum of responses at the cellular and plant levels is replaced by the enormous diversity of species (350,000 species of vascular plants), which respond to environmental conditions. Therefore, in Part IV of this book, the temporal dimensions (Chap. 17), spatial dimensions (Chap. 18) and biological interactions of plants (Chap. 19) are discussed. The numerous factors that determine biodiversity and the effects that biodiversity have on the functioning of ecosystems are summarised in Chap. 20.
Understanding of plant ecology at all levels, from cells to plants to communities and ecosystems, is the fundamental prerequisite for addressing pressing global problems related to plants and ecosystems. With respect to food security, one of the most relevant aspects is global change, which is also discussed by the wider public. Knowing answers to the question of how the biosphere reacts to direct and indirect anthropogenic impacts triggered by land use, changes in land use and changes in climate caused by the use of fossil energy will help us to develop strategies to sustainably manage the Earth’s terrestrial ecosystems. Thus, plant ecology—including aspects of agriculture and silviculture—plays an important and even vital role in providing scientific information for decision makers in policy and society to address questions concerning ecosystem health and human well-being. Therefore in Part V, global biogeochemical cycles and ecosystem services are introduced (Chap. 21). The complex interactions between global ecosystems and their global environment are presented using dynamic global vegetation models in Chap. 22. The future challenges for terrestrial ecosystems at times of global change and potential solutions in terms of international agreements are discussed in Chap. 23.

The distribution of a plant species depends on different environmental factors. The actual distribution range (shown in blue) is significantly smaller than the potential area of distribution that is reached without competition—for example, at the extreme limits of flowering or at the boundaries of a positive carbon budget. Human interventions, particularly in agriculture, interact with these natural filters and can expand the spatial distribution of a species tremendously. In the example shown, temperature is the dominant factor. The limits of distribution change according to the species. The historical dimension of distribution is encapsulated in the present geographical range
When we are dealing with processes and responses on different scales, ranging from the cell to the Earth’s surface, it becomes apparent that over time, scientific disciplines working on these different scales have developed their own sets of definitions and terminology. When we move from the cell to the global biosphere, the precision of interpretation decreases because the system’s complexity increases. With each new scale, the initial processes continue but new interactions are added. This requires simplification of increasing complexity to the level of a few driving variables that we, as human beings, are able to understand. In parallel, a change in terminology happens; thus, the use of words also changes with scale. For example, the level of detail that is known and called a “control factor” or “regulatory factor” at the genetic level is not comparable to a control factor driving processes at the ecosystem level. This is a fact we have to accept and keep in mind when reading this book, which aims to link and span all of these scales of plant ecology.
Acknowledgements
It has been a pleasure, but also a great task, to write the second edition of this book, at the cost of what everyone lacks the most: time. Therefore, our families deserve our main gratitude, since they have given us the time and the support to carry out this work. Moreover, without the support of and discussions with many friends and colleagues, it would not have been possible to finish this revised second edition of the book. Thus, the authors express their thankfulness to Werner Eugster (Zurich), who critically reviewed Chap. 9; Eckhard George (Grossbeeren), who critically reviewed Chap. 11; Neringa Mannerheim (Zurich), who commented on Chaps. 10 and 12; Fritz Schweingruber (Birmensdorf) for his input into Chap. 12; and Kevin Solarik (Edmonton), who reviewed and linguistically improved Chaps. 17 and 18. Cornelia and Axel Paulsch (Regensburg) provided the description of the Convention of Biological Diversity in Chap. 23; Jed Kaplan (Lausanne) provided high-resolution maps, and Matti Barthel (Zurich) provided input into Part V. Special thanks go to Anna Gilgen (Zurich), who not only inspected equations and references but also checked the comprehensibility of Parts II, III and V in a tireless fashion and kept track of the many big and small tasks related to the writing of this second edition. We also greatly acknowledge the technical assistance of Barbara Lühker (Heidelberg) and Annett Boerner (Adelaide) for the first edition. Special thanks also go to Alexandra Böhminghaus (Freiburg), Ursula Ferrera (Bayreuth) and Sabina Keller (Zurich) for their technical support to complete the second edition.