Chapter 11’s discussion of bone fracture suggested that, given the strong structural influence on skeletal element fracture patterns, different actors are likely to produce similar break morphologies. Other taphonomic traces, usually bone surface modifications, often offer better resolution concerning the actor or actors involved. This chapter introduces modifications inflicted on bone by carnivorous mammals, including humans, and by crocodiles, the only reptile group for which bone modifications have been recorded. Carnivores consume blood, muscle, body fat, internal organs, fat and red marrow in pore spaces of cancellous bone segments, and yellow marrow in endosteal spaces. As they acquire these tissues, they modify and even consume bone tissue. The hyena family has evolved massive dentition, jaw musculature, and stomach acid that enable them to consume and digest bone, assimilating the collagen and fat in such tissue.
In the 1980s, definitive traits of carnivore tooth marks were distinguished from marks made by hominin tools. With a few notable exceptions (Binford 1981; Haynes 1980a), the first wave of literature on carnivore bone surface modifications were made by zooarchaeologists focusing on Plio-Pleistocene archaeofaunas . South American and Australian researchers working with Upper Pleistocene and Holocene archaeofaunas later contributed data from different carnivore communities.
This chapter begins with a general review of mammalian carnivores ’ processing vertebrate bodies, including carcass and bone consumption sequences . It then outlines specific effects of different carnivore families, relating these to their anatomy and the tissues they habitually consume. It ends by introducing factors that influence in-place destruction of skeletal elements by carnivores vs. their transport of elements to other locales, a topic discussed again Chaps. 19, 20, and 21.
For zooarchaeologists dealing with archaeofaunas from medieval Europe or plantation sites in the pre-Civil War United States, the effects of hyenas, wolves, and big cats might seem irrelevant. However, domestic dogs, especially those spurred by humans’ practice of letting them fend for themselves, have been ubiquitous and exceptionally motivated bone processors in human settlements for much of the Holocene. Zooarchaeologists working when and where dogs may be present need to recognize signs of carnivore impacts on their bone assemblages. Furthermore, if researchers plan to use element frequencies in their analyses, they must construct the case for excluding destruction by dogs as a major determinant of assemblage structure, citing bone surface modifications and relevant contextual evidence. The simple absence of dog remains in an archaeofaunal sample is not proof their absence as a taphonomic agent, since disposal of dog carcasses is often culturally structured and may result in discard away from food debris.
Focusing as it does on the actor-effector level, this chapter will not address the controversies over the behavioral and ecological meaning of tooth mark frequencies in archaeofaunas . Patterns of aggregate archaeofaunal data will be taken up in Sect. 12.5.
12.1 Carnivore Effects on Prey Bodies and Bones: An Orientation
With the exception of vultures (Chap. 13), few vertebrates subsist entirely by scavenging. Vertebrate carnivores may hunt living animals and scavenge dead ones. Spotted and striped hyenas, popularly considered scavengers, actually hunt and scavenge. Kruuk (1972) found that quite a few lion “kills” were in fact hyena prey that were subsequently appropriated by lions. Without witnessing a kill, one cannot assume beforehand whether a predator obtained prey animals by hunting or by scavenging, which makes a term for obtaining an animal body, without stipulating how, very useful. I have used carcass acquisition and carcass acquisition locale (Gifford-Gonzalez 1993) as neutral terms that imply nothing about how an actor, human or non-human, took possession of an animal body, but simply that it did so.
Carnivores have a somewhat stereotyped sequence of consuming intact carcasses of larger prey, and the marks they leave will generally be parallel across various taxa, although the intensity and placement of marks may vary according to contextual factors and predator anatomical traits, as will be seen in the next section of this chapter. For instance, when scavengers consume remnants of a partially eaten carcass, the initial condition of the carcass upon encounter influences their handling tactics.
- 1.
Modification to bone surfaces as marks inflicted during kill and consumption. These were formerly called “tooth marks ” or “gnawing marks,” but greater specificity is now used.
- 2.
Destruction of entire bone elements or of segments of elements during consumption. This has been termed “carnivore attrition” or “carnivore ravaging ” in the North American literature.
- 3.
Transport of bones individually or in body segments away from the acquisition locale, with their accumulation in other locales (burrows, caves, cliffs, trees).
These three types of carnivore effects parallel those of humans, who modify bones during killing, dismemberment , and nutrient extraction, destroy some elements or segments of elements while processing them, and selectively transport body segments to other locales. Humans also directly modify bones with their teeth , as will be outlined in this chapter. Understanding the key differences – or sometimes the lack of them – between nonhuman carnivore and human effects on bone is thus important to assemblage analyses in regions inhabited by larger carnivores as well as hominins.
In early works on taphonomy, carnivore impacts were seen as a major “bias” affecting bones as they moved from life context to fossil context. Modification and deletion of skeletal elements or segments by carnivores is undeniable, but it is more useful to focus on the evidence added by such processors (Chap. 3). Tooth marks and other traces of carnivore action on bone are “trace fossils ” (Bishop 1975), reflecting ecological relations between species. Likewise, carnivore bone accumulations do not just represent deletion of prey animals’ bones from where they died: these specimens offer information on predator behavior in ancient landscapes. If context and other taphonomic modifications indicate that humans originally generated an assemblage that carnivores then modified, this reveals interactions of at least three species – prey animals, humans, and carnivores . To paraphrase Andrew Sillen (1989:228) again, carnivore bone modification is not the “mist on the window” into the past, but is instead part of the view.
12.1.1 Environment, Ecology, and Behavior: Relation to Carnivore Bone Processing
There is no such thing as a typical carnivore, even among terrestrial mammals. Canids, felids, hyenids, ursids, mustelids, herpestids (mongooses and relatives), and Australian marsupial carnivores differ in their predatory and feeding adaptations and thus in their impacts on skeletal elements . Modern studies also indicate that mammalian carnivore species are extraordinarily flexible in their behavior as they respond to local and regional ecological variability. Prey species choice, killing methods, and carcass handling can vary substantially within one species. This especially applies to the extent to which predators consume bone. Actualistic research has shown that behaviorally flexible carnivores respond to changes in local conditions by changing their transport and consumption behavior, which in turn influences the osseous record of their activities. Kruuk (1972) and Blumenschine (1989) report demographically driven behavioral variability for spotted hyenas of the Serengeti-Ngorongoro region of northern Tanzania. In Ngorongoro Crater, where spotted hyenas were densest, they hunted in packs, engaged in hostile interactions with other hyena groups, and tried to drive lions from their kills. In other areas, spotted hyenas were fewer, lived in small social groups, and obtained more food by non-confrontational scavenging. In a detailed 40-year analysis, Faith and Behrensmeyer (2006) document a major decrease in carcass and element frequencies in the Amboseli National Park landscape as a lion-dominant ecosystem, with an abundance of drought deaths, was supplanted over three decades by a hyena-dominant carnivore community with some lions, cheetahs, and jackals.
It follows that, to evaluate carnivores ’ role in modifying an archaeofaunal sample, it is essential to have some grasp of the carnivore community extant when and where the assemblage formed (Delaney-Rivera et al. 2009). If hominins lived in animal communities with high numbers of wild carnivore species and considerable competition, bone consumption and transport effects may be stronger than among the same species under less densely packed conditions (Gidna et al. 2013). Likewise, unprovisioned scavenger dogs in human settlements, as opposed to well cared for working dogs or pampered pets, may attack bones differently. Such knowledge, if at all possible to obtain, permits a more discerning view of carnivore modifications in an archaeofaunal sample. To gain a sense of carnivore community structure and possible levels of motivation to move and consume bones, zooarchaeologists studying recent archaeofaunas can use historic records or data from prior archaeological analyses. At deeper time depths, assessing carnivore communities and their effects on carnivore bone modification is more challenging but zooarchaeological analyses demonstrate it is not impossible. Paleontological reports permit assessment of predatory adaptations and as well as the functional anatomy and locomotor and feeding patterns of extinct carnivores (e.g. Delaney-Rivera et al. 2009; Van Valkenburgh 1988). Marean (1989) assessed the role of saber-toothed machairodont cats in the Olduvai paleocommunity, arguing that analogies with modern carnivore communities neglects the unique scavenging opportunities these predators may have offered hominins. Stiner (1994) began her analysis of Italian Middle Palaeolithic archaeofaunas from caves by reviewing carnivore taxa documented in earlier research, then assessing modern conspecifics’ or close relatives’ cave use and effects on bone.
Although zooarchaeologists’ and taphonomists’ observations of wild and captive animal were intended to elucidate bone modification, they have also clarified the roles of carnivore feeding strategies and of bones in ecosystems (Blumenschine 1989; Blumenschine et al. 1994; Haynes 1980b). As such, they have bridged between biological approaches to animals in landscapes and zooarchaeological and paleontological analysis.
12.1.2 Relation of Consumer Size to Size of Affected Carcass or Bone
The bone modifications described in this chapter reflect circumstances that leave some residual bone for zooarchaeological analysis. Just as the morphology of breakage to fresh bone is to an extent governed by the relation between the force an actor can apply and the level of force an element can withstand (Chap. 11), so, too, bones’ survival varies according to a similar interaction of size relations. When a wolf captures a lemming, it makes short work of eating the entire animal. The action of the wolf’s strong jaws and stomach acids reduce the lemming’s bones to small fragments or completely destroy them. The same wolf feeding on an adult deer or caribou handles the carcass according to a sequence that maximizes recovery of nutritious tissue while minimizing energy expenditure and risk of injury while dealing with a body larger than its own. This case will leave more skeletal remains, some maybe scattered or transported, some bearing traces of the wolf’s feeding behavior. If the same wolf were to acquire a bison carcass, it would follow similar cost-benefit strategies as in handling deer or caribou, but with relatively diminished ability to disarticulate body segments or to consume bones, perhaps resulting in a less dispersed skeleton.
12.1.3 Carnivore “Ravaging:” A Plea for a More Objective Terminology
Before turning to carnivore modifications to bones, I want to argue for a more objective descriptive terminology. Following Binford (1981; Binford and Bertram 1977), several influential North American zooarchaeologists (e.g. Lyman 1987; Marean and Spencer 1991) have adopted the phrase “carnivore ravaging ” to describe destruction of bones and bone segments by nonhuman meat consumers. By “ravaging,” Binford meant the typical patterns of carnivore destruction of bones. This is an unfortunate term because of its common connotations and its implicit decoupling of carnivore effects on bone from their ecological context. The verb “to ravage” means to pillage, ruin, annihilate, destroy, ransack, lay waste to, devastate, or plunder (Merriam-Webster.com 2008), sometimes used metaphorically, as in “the ravages of time.”
Calling what carnivores do to bone as an integral part of their behavioral ecology “ravaging” is both inaccurate and parochial. Though Binford probably never intended to divorce carnivore impacts on bones from their ecological contexts, “ravaging,” suggests an unproductively anthropocentric approach. It implies that carnivore action is the “mist on the windscreen” of archaeofaunal analysis, to use Sillen’s terms again, rather than “part of the view.” Less pejorative phrases, such as, “carnivore bone reduction,” or “carnivore processing,” could be used with equal ease and greater scientific and biological precision . Given the use of “handling time ” in behavioral ecology , “carnivore handling” is another alternative.
12.2 Carcass Consumption Sequences
- 1.
Anal region, belly, and internal organs.
- 2.
Hindquarters: rump and upper hind leg.
- 3.
Back ribs, including cartilage.
- 4.
Forequarters: shoulder and upper foreleg.
- 5.
Head and neck muscle.
- 6.
Lower limb bones.
According to the condition of consumer and prey and levels of intra- and interspecific competition for nutrients, a carnivore will go through part or all of this sequence before abandoning the carcass.
12.2.1 Bone Reduction by Canids and Hyenids
- 1.
Least dense and most porous bones, cartilaginous ends and then bones of ribs, vertebrae, scapulae, innominates, and other bones.
- 2.
Slightly more dense cancellous epiphyses of long bones.
- 3.
Denser compact bone elements enclosing edible soft tissues.
The same factors as influenced carcass consumption will affect how thoroughly a carnivore pursues this sequence.
12.3 Carnivore Effects on Skeletal Elements and Segments
Living carnivores that can most intensively modify skeletal elements are members of the zoological families Canidae (dogs and foxes) and Hyaenidae (hyena species). Canids and hyenid long bone consumption is outlined by body segment below (Binford 1981; Binford and Bertram 1977; Haynes 1980a, b).
12.3.1 Skull
As large and relatively thick-walled bone units, crania present a major challenge to potential consumers. Crania that fit into a consumer’s jaws can be punctured or collapsed by static loading . Blumenschine (1986) and others noted that larger ungulate crania are left more or less intact in the Serengeti ecosystem, even after hyena feeding. If carnivores can accomplish any cranial reduction, it is usually through the facial region. Horwitz and Smith (1988) note that striped hyenas habitually removed facial bones from human crania dug from graves, and that some were reduced to fragments. In ethnoarchaeological site surveys along northeastern Lake Turkana shorelines, I encountered two adult human crania that had all ethmoid, sphenoid, and basicranial bone removed and hyena-size tooth marks near the break margins. Variations in the motivation of hyenas to break down braincases may reflect ecologically based differences in food availability: the low productivity environments of the Negev and Lake Turkana offered fewer opportunities to obtain protein and fat-rich tissues such as offered by the brain (Stiner 1994), and hyenas may risk tooth damage to obtain them in these situations versus the higher productivity Serengeti (Van Valkenburgh et al. 1990).
In ungulate species and in humans, mandibles readily detach from the cranium (Chap. 19). Roots of mammal teeth are embedded in a blood- and fat-rich tissue, called “pulp” in dentistry, within the dentary bone. Grazers and browsers with high-crowned teeth that erupt over years have deeper dentaries and more pulp. Carnivores gnaw the angle of the mandible or the underside of the dentary to open the pulp cavity. This leaves the dental row intact and may not damage the ascending ramus (Binford 1981).
12.3.2 Vertebrae
Vertebrae are some of the more delicate elements in the skeleton, being substantially reduced by consumers of sizes smaller than the prey animal and completely destroyed by consumers of equal or larger body sizes. Captive hyenas consumed nearly 100% of sheep vertebrae in captive feeding trials (Marean and Spencer 1991; Marean et al. 1992). Similar results were reported by Binford et al. (1988) monitoring wild hyena bone processing of equal and slightly larger animals. Brain (1981) reports that cheetahs, not habitual bone consumers, nonetheless reduce vertebral segments of baboons and small gazelles, as can leopards observed in captive feeding trials. Transverse and spinous processes of vertebrae often display gnawing marks resulting from attempts to remove the attached muscle tissues. The relatively dense zygapophyses, the small articular facets on the fore and aft of each vertebral arch, may remain.
12.3.3 Ribs
Ribs contain red marrow and are attractive to carnivores . They also serve as anchors for complexly layered thoracic and abdominal muscles, and their proximal ends underlie the muscle groups running longitudinally down the spine. Consumers may damage ribs as they feed on those muscles. Carnivores normally reduce ribs attaching to the sternum via a cartilage link from the sternal end, or, if floating ribs, from the ends terminating in cartilage. Heads of ribs may be chewed if dislocated or disarticulated from thoracic vertebrae.
12.3.4 Shoulder and Pelvic Girdle
Blades of scapulae and innominates, with cartilage plates at their extremities and multiple muscular attachments, are usually gnawed inward from their zones of cartilage and muscle attachments. Areas typically displaying tooth marks are the vertebral border of the scapula, and in the innominates, the ischial tuberosity, the iliac crest, and sometimes the pubic symphysis (see Binford 1981: Fig. 3.36).
12.3.5 Long Bones
Trabecular tissues of the least dense epiphyseal ends of the bone are consumed first, using incisors, canines, and tongue to gouge and scoop out cancellous tissue, producing a cylinder of the compact diaphyseal bone. The carnivore attempts to collapse the cylinder by compressing it at more or less right angles between its carnassial teeth (upper P4 and lower M1). If the cylinder does not collapse, the actor orients it in parallel to its carnassial teeth and presses down to puncture the shaft, repeating the action to open a “channel” in parallel to the long axis of the bone, driving off chips of bone in the process. Canids may stabilize the shaft between their front paws while doing this. Once the cylinder is sufficiently weakened by channeling, the actor collapses it by compression and gains access to the marrow in the endosteal cavity, leaving long, channeled, and flaked splinters.
Binford (1981:51) described another kind of long bone reduction, “chipping back,” a variant of channeling, in that the bone is held in the same orientation and carnassials are applied. The teeth may be placed against a ridge or process on the cylinder, and the carnassials pressing on the cortical bone remove small flakes in a kind of pressure flaking. Bone fragments and small, dense bones may be swallowed whole and subject to stomach acids. In canids, undissolved fragments pass through digestive track and are excreted in the feces. Hyenas vomit bone not fully digested.
12.3.6 Carpals, Tarsals, Metapodials, and Phalanges
In ungulates, the metacarpals and metatarsals are long bones, and they are treated as such by non-human carnivores : gnawed at epiphyses, especially the less dense distal ends, and diaphyses collapsed for yellow marrow within the diaphysis . Toe bones are often nibbled away for the marrow they contain and the fat in their cushion pad. If carnivores carry prey limbs away from the acquisition site, carpals and tarsals may “ride” with the more nutritionally rewarding long bones with which they are tightly articulated (Binford 1978; 1981, see Chaps. 18 and 19). Hyenas are reported to swallow, digest, and/or regurgitate dense bones of the carpal and tarsal joints (Marean 1991).
12.4 Characteristic Marks of Carnivore Processing
Carnivore marks inflicted on bones are made by teeth , by polishing with the tongue, or by stomach acids, after bone fragments have been ingested. These typical marks were first studied in canids , and then the effects of spotted hyenas were documented. Key works include Binford (1962, 1981), Haynes (1980a, 1983), Shipman and Rose (1983), and a critical comparative review by Lyman (1987).
12.4.1 Tooth Marks and Other Carnivore Modifications
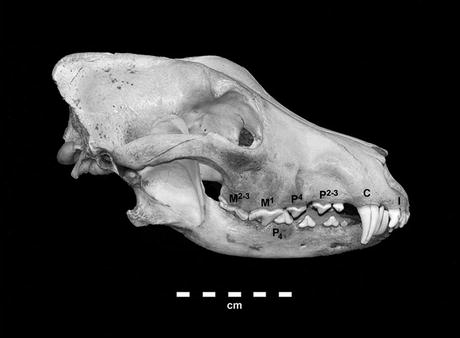
A wolf cranium and mandible, showing the incisors (I), canine (C), anterior premolars (P2-3), carnassials (lower P4 and Upper M1), and posterior molars. While this dental arrangement differs from one zoological family to another in the precise numbers of teeth, it is generally similar in all placental carnivores and has strong parallels in marsupial carnivores. (Photo by the author)
- 1.
Tooth pits are made on cortical bone by the anterior premolars or carnassials. They are relatively small marks, often triangular or diamond-shaped when seen from above (Fig. 12.2), but if made by individuals with worn and broken teeth , they may be rounder or irregular. They are often associated with tooth scores.Fig. 12.2
Cave bear (Ursus spelaeus) patella from Pleistocene deposits in Pod Hradem Cave, Czech Republic, showing smaller, sub-triangular tooth punctures and furrowing from the edge of the articular surface. Tooth marks are within the size range of deciduous wolf (Canis lupus) teeth. Scale bar is 1 cm. (Photo by Robert H. Gargett (1996: Fig. 6.18), used with permission of author and University Press of America)
- 2.
Tooth scores are grooves usually produced on cortical bone by anterior premolars or carnassials. They are made as a tooth cusp drags over the compact bone surface, from the pit initially made by the cusp on compression (Fig. 12.3). Longer scores usually curve slightly, and scores are U-shaped in cross-section compared to cut marks (Chap. 14). Scores may show downward crushing of bone into the groove and lack linear striations typical of stone tool cut marks (Fig. 12.4).Fig. 12.3
Fossil bone surface from the Nihewan Basin, Hebei Province, People’s Republic of China, showing the relation of the initial pit made by a tooth cusp to a score resulting from the tooth being dragged across the bone surface. (Photo by Don Harris of a specimen donated to the author by Dr. Wei Qi, 1989)
Fig. 12.4Heavily dog-gnawed bovine bone (Bos taurus), showing tooth scores over the surfaces, with chipping-back and rounding of the distal end of the bone. (Photo by Don Harris of experimental specimen collected by the author)
- 3.
Punctures are holes punched through thin overlying cortical bone into cancellous tissue or into an underlying cavity. Punctures are usually made by canines or premolar cusps and are roughly oval or circular. They often display downward displacement of the cortical bone layer into the hole (Fig. 12.5).Fig. 12.5
Bovine (Bos taurus) proximal femur from Site 105, a modern pastoralist encampment near Koobi Fora, East Lake Turkana, Kenya, showing hyena-sized carnivore tooth puncture on femoral head. (Photo by Don Harris of specimen collected by the author in September 1973)
- 4.
Flaking of cortical bone , especially long bones, results from static loading homologous to lithic pressure flaking, as a carnivore grips compact bone between the cusps of two opposing teeth . If one such compressing cusp lies on an acutely angled edge of broken bone, the bone fails, driving off a flake from the outside of the bone into the endosteal cavity.
- (a)
If teeth are placed along a crack parallel to the long axis of a long bone, or along the edge of a flat bone such as a scapula, and if the compression is repeated, a continuous line of small flake scars may develop, forming a scalloped edge (Fig. 12.6). Binford called this crenulation . Flakes are normally neither stepped nor hinged and lack crushing at the point of percussion that is typical of human hammerstone impacts (Chap. 14).Fig. 12.6
Shaft fragment of a mule deer (Odocoileus hemionus) femur, showing crenulation by carnivore tooth action (tooth size in range of coyote, Canis latrans). Break surfaces and edges also display rounding and polishing resulting from licking. (Photo by Don Harris of a donated specimen from Stanislaus National Forest in the author’s lab)
- (b)
If the compact bone cylinder is clamped between teeth at an end, and a section is then levered upward with the actor’s neck muscles, a flake scar oriented parallel to the long axis of the bone is driven off. Binford (1981:51–54) termed this chipping-back and stated these scars more commonly display stepping (Fig. 12.7).Fig. 12.7
A bovine (Bos taurus) femur shaft fragment heavily gnawed by dogs, showing tooth scores over the surface, with chipping-back and rounding and polishing of the distal end of the bone. Greater light reflectance at left is due to the polishing action of the dog’s tongue. (Photo by Don Harris of experimental specimen collected by the author)
- 5.
Furrowing is damage to cancellous bone caused by repeated scraping of canines or premolars across the trabeculae , producing high and low rows, or furrows (Fig. 12.8). Furrowing results from reiterated gnawing similar to that producing crenulation in cortical bone but furrows develop gouges in trabecular bone instead.Fig. 12.8
Bovine (Bos taurus) proximal humerus from Site 105, a modern pastoralist encampment near Koobi Fora, East Lake Turkana, Kenya, showing hyena-sized carnivore tooth furrows on humeral head, with some compact bone depressed into the underlying cancellous tissue (Photo by Don Harris of specimen collected the author in September 1973)
- 6.
Scooping out is the removal of cancellous bone from the spongey bone within a diaphysis at either end of a long bone. The actor pushes the drags incisors into the cancellous tissue and scrapes with the teeth , licking out the broken-down cancellous tissue. Canids using this technique can sometimes invade the marrow cavity (Fig. 12.9).Fig. 12.9
The same heavily dog-gnawed bovine femur (Bos taurus) as in Fig. 11.4, showing scooping-out of the cancellous tissues at the distal end, plus chipping-back, rounding and polishing of the compact bone at the bone’s distal end. Greater light reflectance is due to the polishing action of the dog’s tongue. (Photo by Don Harris of experimental specimen collected by the author)
- 7.
Smoothing and rounding is produced by repeated licking and grinding of a compact bone fracture surface by tongue and teeth. It may be mistaken for tool use or other abrasion (Figs. 12.6, 12.7, and 12.9).
- 8.
Lamellar and cortical bone loss, pitting, rounding, thinning of bone walls is typical of stomach acid modifications and may be found either in vomited or defecated prey bones of carnivores , depending on the taxon. High frequencies of such bones in an area may reflect where carnivores have habitually defecated near dens or where dogs were confined (Fig. 12.10).Fig. 12.10
Rodent mandible that has passed through the digestive system of a captive margay (Leopardus wedeii), showing loss of lamellar bone, thinning and rounding of bone walls. Each bar of dashed line in upper left is 1 micron. (Unpublished photo by Peter J. Andrews, used with his permission)
Large carnivores will reduce large animals’ epiphyseal ends first. Only when driven by the need for lipids will most large carnivores other than spotted hyenas attempt to collapse diaphyses. Figures 12.4 and 12.7 show the unsuccessful efforts of Labrador retriever and German shepherd dogs to collapse limb shafts of cattle femora, despite much gnawing, and wolves appear to calibrate their efforts to bone sizes they can break without too much effort and risk (Binford 1981). A major difference between larger ungulate skeletal assemblages processed by large carnivores and those of the same sized prey processed by humans is in the reduction versus non-reduction of long bone epiphyses and other elements with lower densities of bone mineral. In the simplest case, assemblages processed by carnivores would be characterized by a lack of bones composed mainly of cancellous tissues, missing long bone epiphyses, the presence of diaphyseal cylinders, or collapsed fragments of them, and many fragments bearing some tooth marks . Samples produced exclusively by humans will preserve delicate elements and long bone epiphyses and display traces fragmentation by percussion to the shafts. Matters are not usually so straightforward in real life. Some assemblages of ancient bones show the effects of carnivores and of hominins, and much basic research has been aimed at clarifying such “multi-agent” assemblages, an area that will be discussed in more detail in Chap. 17.
12.5 Differing Effects of Carnivore Taxa: Surface Modifications and Bone Destruction
Although a general relationship exists in the amount of damage a consuming carnivore can cause based on its size relative to that of the consumed prey, different families of carnivores vary in their damage to bones. The following section summarizes the effects of members of major taxonomic groups.
12.5.1 Canids
Wild and domesticated dogs eat flesh, blood, and internal organs and gnaw bones to obtain nutrients in them. Canids have somewhat less knife-like molar teeth than do felids, and they can more readily gnaw bones without risking tooth breakage than can cats. Young wild canids more than adults gnaw bones as they develop their jaw muscles and erupt their adult teeth. Domestic dogs may gnaw bones more than do wild dogs, partly because they often depend on leavings from human meals, and partly because they may have been selected by humans to continue to show “infantile” behaviors into adulthood, such as being friendly, not overly predatory, etc. They may therefore be “stuck” at the gnawing stage of development that adult wild canids leave behind.
Canids favor cancellous bone of vertebrae, ends of long bones, the innominates, and ribs, to gain access to fat-rich neural tissues of the spinal cord and blood cells in the spongy bone , as well as to endosteal marrow in long bones. Canid feces may contain bone fragments displaying erosion from stomach acids (Fig. 12.10).
Wolves are the best-documented canid species that transporting bones of larger prey to lairs to nourish the young (Stiner 1991). However, recent studies of two South American fox species, Dusicyon paeus and D. griseus, have shown that some prey species’ bones are also accumulated in their dens in rock shelters (Mondini 1995).
12.5.2 Felids

Puncture marks made by the canine and premolar of a puma (Puma concolor) in a scapula of a female mule deer (Odocoileus hemionus), killed by a puma on the University of California, Santa Cruz campus. (Photo: Don Harris of bone in UCSC Anthropology Teaching Laboratories, UC Santa Cruz)

Femur from same female mule deer (Odocoileus hemionus) killed by a puma as in Fig. 12.11, showing shearing across greater trochanter associated with detachment of the hind leg from the hip socket (arrow). Animal killed on the University of California, Santa Cruz campus. (Photo: Don Harris of bone collected by UCSC Anthropology Teaching Laboratories, UC Santa Cruz)

Paired marks of the posterior surface of canines of Homeotherium sabertooth cat on tibia of a deer (arrows) from Nihewan Basin, Hebei Province, Peoples Republic of China. This probably resulted by a sabertooth catching the leg of a living deer in its jaws to capture it, rather than from consumption. (a) Close-up of the left mark; (b) Close up of right mark (arrows). (c) Paired tooth marks on other side of the tibia. (Photos by Don Harris of specimen courtesy Dr. Wei Qi, composite by the author)
South American zooarchaeologists and taphonomists have established that pumas (Puma concolor), one of two large American cats, can gnaw bones and transport body segments to dens. In addition to marks illustrated in Figs. 12.11 and 12.12, pumas can inflict pitting, scoring, scooping-out, crenulation, and long bone fractures on prey bones as large as the guanaco (Lama guanacoe), which weigh 53–64 kg (Borrero et al. 2005). Mondini and Muñoz (2008) summarize findings from a variety of South American habitats and discuss ecological factors that may structure variations in pumas’ bone-consuming behavior.
Based on their tooth form and postcranial anatomy, extinct saber-toothed cats such as Smilodon, Dinofelis, and Homotherium are now thought to have preyed on large, slow-moving prey. Increasing evidence exists for diversity in various species’ handling of bone. Based on comparison tooth morphology and dental microwear in modern large cats and Smilodon fatalis, Van Valkenburgh et al. (1990) established that Smilodon probably had even less dental contact with bone than do cheetahs. Analyzing element frequencies and bone surface modifications of the paleontological archaeofauna from Friesenham Cave, Texas, Marean and Ehrhardt (1995) inferred that Homeotherium probably transported limbs of immature proboscidean prey to its lair, removing flesh with its serrated teeth . Prey species and body segment representation in South African australopithecine cave archaeofaunas diverge from what would be expected from that typical of Africa’s only prey-transporting cat, the leopard, also suggesting diversity in ancient felid ecology (de Ruiter and Berger 2000; Pickering et al. 2004).
12.5.3 Hyenids
Four hyena species of Hyaenidae live in African and Asia today and formerly had broader ranges in Eurasia. Three have massive jaw muscles, stout, bone-cracking teeth with rounded cusps, and stomach acids capable of thoroughly dissolving bioapatite . The spotted hyena (Crocuta crocuta) can digest bones of animals up to rhinoceros size (Kruuk 1972). Spotted hyenas living in larger groups hunt socially, while solitary animals or those small groups emphasize scavenging. In areas with denser human populations, they scavenge human refuse and sometimes dig up burials. Such disparate foraging strategies are reflected in hyenas’ effects on bones in the landscape, as carcasses rapidly disappear in high density areas but remain in lower density zones.
In habitats not heavily altered by humans, striped hyenas (Hyaena hyaena) hunt porcupines, rodents, and other smaller prey and scavenge larger animals, while scavenging refuse and human burials in heavily settled areas (Horwitz and Smith 1988; Kuhn et al. 2009; Lotan 2000; Monchot and Mashkour 2010). The brown hyena (Parahyena brunnea) was formerly widespread in southern Africa but is more sensitive to human habitat alterations than are spotted hyenas. Brown hyenas hunt small game, scavenge animals killed by other predators, hiding body segments under bushes, or transporting them to dens (Mills and Mills 1978). They can reduce the long bone ends of medium- to large-sized artiodactyls. Scott and Klein (1981) report a fossil brown hyena den, and Kuhn et al. (2009) report modern dens in the Rietvlei Nature Reserve, Namibia, as well as summarizing the literature on effects of three species.
Hyenas do not pass large bone fragments through their digestive tracts. They dissolve bone fragments into a calcium-rich “porridge” in their stomachs with extremely strong stomach acids and vomit out any larger fragments. Their feces are often pure white after drying because of the dissolved bone. Therefore, in contrast to feces of canids, hyena excrement should contain no bone fragments recognizable as such.
Marean , Blumenschine and coworkers (Marean and Spencer 1991; Marean et al. 1992) assessed the impacts of captive spotted hyenas, in experimental feeding trials with sheep-sized skeletal elements . They offered one and four hyenas, depending upon the trial, two types of bone samples. The first were intact bones stripped of most of their muscle and other external tissues but with all nutritional tissues in them, including yellow marrow . The second were intended to mimic elements processed by hominins, that is, not only stripped of meat but also broken open with hammerstones and marrow extracted. The latter was aimed at eliciting traits of so-called “dual-patterned” assemblages in which scavengers consumed hominin bone refuse. In the first set of experiments, hyenas consumed epiphyses, collapsed diaphyses, and consumed the yellow marrow in the endosteal cavity (Figs. 12.5 and 12.8). In the second, they consumed epiphyses but did not gnaw the diaphyses, as no marrow was to be gained by doing so. Researchers concluded that the presence or absence of mid-shaft carnivore tooth marks was a reasonable index of the order in which hominins and bone chewing carnivores had access to skeletal elements . Domínguez-Rodrigo and colleagues (Domínguez-Rodrigo 2003; Domínguez-Rodrigo and Barba 2006) critically assessed these arguments, and the controversy over the meaning of marks on Olduvai bones has continued (e.g. Blumenschine et al. 2007).
12.5.4 Ursids
Extant bear species vary considerably in their degree of carnivorousness. Ancient species’ feeding habits should therefore be evaluated before assuming they hunted or scavenged. For example, stable isotopic analyses of European Pleistocene cave bears (Ursus spelaeus), combined with biomechanical analysis of their bones, indicates this species was primarily a vegetarian (Bocherens et al. 1990). Stiner et al.’s (1996) discussion of cave bears behavior in Yarimburgaze Cave, Turkey, noted that all living bear species avoid bringing carcasses or body segments to hibernation and birthing dens, with very rare exceptions. Gargett’s (1996) research on the non-archaeological Pleistocene fauna from Pod Hradem Cave, Czech Republic, suggests that cave bears did not gnaw their own species’ bones that accumulated through natural deaths in their hibernation dens. Most tooth marks on Ursus spelaeus bones from Yarimburgaze Cave and Pod Hradem were attributable to canids, probably wolves, and hyenids, probably spotted hyena, which inhabited Eurasia during most of the Pleistocene.

Bovine (Bos) femur gnawed by a captive brown bear (Ursus arctos), showing broad, shallow, furrowed surface of the modifications (Specimen courtesy Gary Haynes, photograph by the author)
12.5.5 Australian Marsupial Carnivores
Before the arrival of Europeans, the only placental carnivore in Australia was the dingo, a feral canid introduced there by aboriginal colonists. A numerous fossil and modern marsupial carnivore species existed on the continent. The best-documented recent marsupial bone collectors and modifiers are the eastern and western quolls, Dasyurus geoffroii and D. viverrinus (Leung 2002; Dela Cruz 2002), and the Tasmanian devil (Sarcophilus harrisi).
Quolls are small predators, weighing 0.85–1.3 kg, which consume insects , ground-nesting birds, and other small terrestrial or arboreal animals. They scavenge and transport parts of animals beyond their own competence to kill, including sheep, to their lairs (Fleay 1932). Based on experimental feeding trials, Walshe (1994) concluded that quolls are unlikely to leave distinctive marks on larger animals’ bones.
Weighing 4–12 kg, Tasmanian devils have massive jaws and are superb scavengers and bone-crushers, capable of consuming all but the largest wombat and kangaroo bones (Guiler 1970; Marshall and Cosgrove 1990). The species was historically confined to Tasmania, but Sarcophilus remains are widespread in Holocene mainland Australian deposits. Tasmanian devils forage on the ground and in trees, taking reptiles, small marsupials, and nesting birds (Dewey et al. 2001). They habitually use dens but, unlike quolls, do not accumulate carcasses or bones in them. Feeding experiments by Sobbe (1990, cited in Walshe 1994) with captive Tasmanian devils documented scores, pits, furrows, and punctures paralleling those inflicted by placental carnivores . However, these marks were left on bones at feeding sites rather than in lairs. Sarcophilus pass bone fragments, occasionally complete feet of small prey, through their digestive systems, thus forming recognizable scat deposits (Marshall and Cosgrove 1990; Walshe 1994).
Walshe (1994:152–68) notes that carnivores much larger than Sarcophilus existed in Late Pleistocene and Holocene Australia, including the thylacine, Thylacinus cynocephalus, and marsupial tiger, Thylacoleo. Thylacine anatomy, and the behavior of the few observed before their historic extinction, suggests that their foraging and bone modifying behaviors may have been more like that of Sarcophilus.
12.5.6 Human Gnawing
Zooarchaeologists have been aware for some time that marks made by human teeth on bones can mimic those of carnivores (e.g. Solomon 1985; White 1992; Binford 1978, 1981; Gifford-Gonzalez 1989; Oliver 1993, 1994). Four actualistic studies, two experimental and two ethnoarchaeological, have clarified these matters considerably. Elkin and Mondini (1996) conducted experimental feeding of identical sets of sheep axial and appendicular bones to humans and Pampa foxes, Pseudalopex gynnocercus (4.5–6.5 kg). More bones in the human experiment were marked gnawing than were bones the fox experiment, but one axial set was apparently completely consumed by the foxes. Both samples included scoring and pits on cortical bone and punctures on cancellous tissues, as well as removal of the ends of bones.
- 1.
Bent ends (fraying)
- 2.
Curved shape at the terminations of thin bones
- 3.
Crenulated edges
- 4.
Double arch punctures on broken edges
- 5.
Triangular, dispersed, and rare puncture marks on bone surfaces
- 6.
Shallow, transverse, or oblique linear marks on bone surface
- 7.
Shallow surface scratches associated with shallow crescent pits made by incisors

SEM image of irregular human tooth pits on the pubic ramus of a giant pouched rat. (Micrograph from Landt (2007:1635, Fig. 3) of ethnoarchaeological bone sample, used with the permission of the author and Elsevier)

Adult caprine radius and metatarsal, showing tool-aided fracturing distally and crushing damage by human mastication to proximal ends, from Dassanetch ethnoarchaeological bone sample collected by author. (Photo by author)
Landt introduced useful distinctions, including that the extent of human tooth marking of bone varied with the size of the prey. Bones of smaller prey such as pouched rat and porcupine showed larger human tooth marks than did those of blue duiker, a small forest antelope or very small murid mice, which he relates to their mode of consumption (Landt 2007:133, 137). Landt (2007:1637) stresses that, “not only are human tooth marks on small animals likely to be confused with those of small canids on similarly sized remains, but the size of the mastication damaged prey is an important variable that needs to be controlled in future research.” This message is reinforced by Martínez (2009) and by Delany-Rivera et al. (2009), whose research included experimental human gnawing effects on bovine ribs (see Can Tooth Marks Distinguish Carnivore Taxa or Size? below).
As might be expected, chimpanzees and baboons produce similar mastication marks on bone to those made by humans, so in contexts where other primates might be present, diagnosis should always involve contextual evidence (Pickering and Wallis 1997; Plummer and Stanford 2000; Delaney-Rivera et al. 2009; Dominguez-Rodrigo and Piqueras 2003). However, for zooarchaeologists working with later archaeofaunas in other areas the criteria outlined above may help sort human impacts from those of smaller carnivores (but see Can Tooth Marks Distinguish Carnivore Taxa or Size? below).
To sum up, some human tooth marks resemble some carnivore tooth marks , especially those of such small canids as foxes, jackals, or smaller dogs (Fig. 12.15). Presence of several of Fernández-Jalvo and Andrews’s eight human dental modifications, as well as a lack of other typically carnivore modifications in an assemblage, increases the likelihood that human actors were responsible for the damage. Other forms of contextual evidence, such as cut or chop marks and spatial setting, may also serve to clarify the likely agent(s) (Fig. 12.16).
12.6 Crocodylian Effects on Bone
Crocodiles and their relatives inhabit tropical and subtropical waters around the world. Some exclusively eat fishes, while others actively prey on terrestrial vertebrates and scavenge carcasses from lake or river margins. In the process, they may leave tooth marks on bones. Larger crocodilians take live prey fording rivers, wading into water to drink or forage, and in some spectacular cases, may lunge on to land to seize an animal standing near the water’s edge (Njau and Blumenschine 2006). Two sets of researchers have conducted captive feeding experiments with Nile crocodiles (Crocodylus niloticus), using medium (1.5–2.0 m) to large (>4 m) animals (Njau and Blumenschine 2006; Baquedano et al. 2012). Njau and Blumenschine also collected ungulate bones from the banks of the Grumeti River, where crocodiles take zebra, wildebeest, and other prey during the yearly migrations to the Serengeti Plains.
Njau and Blumenschine concluded that marks made by crocodylian teeth overlap those made by mammalian predators, but that a few distinctive differences existed. Crocodiles do not gnaw bones and only occasionally fracture them with the pressure of their jaws. Their tooth marks are most common on limbs, and the authors report a high rate of marks, plus two distinctively crocodylian marks. The first the authors called a “bisected pit,” in cortical and cancellous bone (Njau and Blumenschine 2006: Figs. 2, 3, and 4). At eruption, crocodylian teeth possess a ridge, or carination, on the mesial and distal edges of each tooth, which produces the distinctive bisected signature. The second were relatively rare j- or u-shaped “hooked scores” (Njau and Blumenschine 2006: Fig. 6). These are inflicted during the so-called “death roll,” crocodiles ’ unique method of breaking up a carcass: one or more predators clamp down on a limb and forcefully roll in the water, producing such marks as bone moves against the teeth. Baquedano et al. (2012:1731–32) also noted microstriations along tooth scores (Fig. 5).
- 1.
Crocodile -created assemblages are composed of primarily complete elements, with minimal fragmentation .
- 2.
These elements exhibit absence of gross gnawing.
- 3.
Crocodile -generated bone assemblages lack disarticulation of complete skeletal units, with the potential of abandoning a large portion of articulated specimens .
Baquedano et al. (2012) disagreed with Njau and Blumenschine that bisected – what they call carinated – tooth marks have a morphology never documented in marks produced by mammalian carnivores , noting that some terrestrial mammal carnivores such as lions have similar canine morphology. They also noted much lower rates of tooth marks on bones, a difference could stem from the two teams’ differing experimental contexts. Njau and Blumenschine ’s (2006) feeding trials involved many animals actively competing for the food items, while the other, captive animal experiment seldom had more than one large, dominant animal feeding, with others sneaking parts but not actively competing. Baquedano et al. (2012) speculate that lack of a free-for-all in their experiment could account for this disparity. They did not observe “hooked scores,” which they note may be a sample size effect , since these were rare (<0.01%) in Njau and Blumenschine ’s sample of 2000 elements, compared to their 198. Persons working with archaeofaunas in which alligator or crocodile impacts are a possibility are referred to these well-illustrated works.
12.7 Can Tooth Marks Distinguish Carnivore Taxa or Size?
- 1.
Tooth pits of various mammalian carnivores and omnivores are not taxonomically diagnostic.
- 2.
A weak relationship exists between the body size of the processing carnivore and tooth mark size, but considerable overlap exists between marks made by different-sized processors.
- (a)
Marks on diaphyses are not reliable indicators of the size of the consumer. Marks on epiphyses and metaphyses are more reliable indicators.
- (a)
- 3.
Some such overlap is explained by consistent inter-taxonomic differences among the tooth impacts of bone-gnawing species.
- (a)
Marks made by smaller canids and other medium sized processors overlap with those made by large felids.
- (b)
Baboons and humans leave a very wide range of tooth marks on bone, overlapping those made by hyenas and lions in size and shape, as well as those of smaller bone gnawers.
- (a)
When attempting to infer the body mass of bone modifying carnivores, tooth mark dimensions should be used in conjunction with patterns of destruction and modification of skeletal elements of different sizes, which may reflect the maximum jaw gapes of the modifiers (see Relation of Consumer Size and Size of Affected Carcass or Bone).
12.8 Carnivore Carcass Dismemberment and Transport
Carnivores often acquire animal bodies some distance from where they prefer to consume them. Prey that are small relative to the predator are transported whole to preferred locations. Larger animal bodies dismembered during the kill or initial consumption may be carried in segments from the acquisition site. Some species’ repeated actions in transporting body segments build up substantial accumulations of bones in one locale. Carnivores’ selective transport and accumulation parallel those of humans and will be treated in more detail in Chap. 19.