The Just So stories published by
Rudyard Kipling at the very beginning of the 20th century are an
imaginative set of tales about origins. Some of the most famous are
those about the phenotypes of animals – How the Leopard Got his
Spots, The Beginning of the Armadillos, How the Camel Got his
Hump. They are written purely as entertaining fantasies but
scientifically they hark back to a century earlier and Lamarck’s
theory of evolution through the inheritance of acquired
characteristics. Kipling’s stories describe how one animal acquired
a physical characteristic – the elephant’s long trunk, for example
– and the implication is that all the offspring inherited that
characteristic, and hence all elephants now have long
trunks.
Kipling was having fun with his stories,
whereas Lamarck was trying to develop a scientific theory. Like any
good scientist, he tried to collect data relevant to this
hypothesis. In one of the most famous examples of this, Lamarck
recorded that the sons of blacksmiths (a very physical trade)
tended to have larger arm muscles than the sons of weavers (a much
less physical occupation). Lamarck interpreted this as the
blacksmiths’ sons inheriting the acquired phenotype of large
muscles from their fathers.
Our modern interpretation is different.
We recognise that a man whose genes tended to endow him with the
ability to develop large muscles would be at an advantage in a
trade such as blacksmithing. This occupation would attract those
who were genetically best suited to it. Our interpretation would
also encompass the likelihood that the blacksmith’s sons may have
inherited this genetic tendency towards chunky biceps. Finally, we
would acknowledge that at the time that Lamarck was writing,
children were used routinely as additional members of a family
workforce. The children of a blacksmith were more likely than those
of a weaver to be performing relatively heavy manual labour from an
early age and hence would be likely to develop larger arm muscles
as a response to their environment, just as we all do when we pump
iron.
It would be a mistake to look back on
Lamarck and only mock. We no longer accept most of his ideas
scientifically, but we should acknowledge that he was making a
genuine attempt to address important questions. Inevitably, and
quite rightly, Lamarck has been overshadowed by Charles Darwin, the
true colossus of 19th century biology – actually, probably the
colossus of biology generally. Darwin’s model of the evolution of
species via natural selection has been the single most powerful
conceptual framework in biological sciences. Its power became even
greater once married to Mendel’s work on inheritance and our
molecular understanding of DNA as the raw material of
inheritance.
If we wanted to summarise a century and a
half of evolutionary theory in one paragraph we might
say:
Random variation in genes creates
phenotypic variation in individuals. Some individuals will survive
better than others in a particular environment, and these
individuals are likely to have more offspring. These offspring may
inherit the same advantageous genetic variation as their parent, so
they too will have increased breeding success. Eventually, over a
huge number of generations, separate species will
evolve.
The raw material for random variation is
mutation of the DNA sequence of the individual; his or her genome.
Mutation rates are generally very low, and so it takes a long time
for advantageous mutations to develop and to spread through a
population. This is especially the case if each mutation only gives
an individual a slight advantage over its competitors in a
particular environment.
This is where the Lamarckian model of
acquired characteristics really falls over, relative to Darwinian
models. An acquired change in phenotype would somehow have to
‘feed-back’ onto the DNA script and change it really dramatically,
so that the acquired characteristic could be transmitted in the
space of just one generation, from parent to child. But there’s
very little evidence that this happens, except occasionally in
response to chemicals or irradiation which damage DNA (mutagens),
causing a change in the actual base-pair sequence. Even these
mutagens only affect the genome at a relatively small percentage of
base-pairs and in a random pattern, so these still can’t drive
inheritance of acquired characteristics in any meaningful
way.
The overwhelming body of data argues
against Lamarckian inheritance, so there’s very little reason for
individual scientists to work on this experimentally. This isn’t
surprising. After all, if you are a scientist interested in the
Solar System, you could choose to investigate the hypothesis that
at least some parts of the Moon are made of cheese. But to do so
would mean that you wilfully ignored the large body of evidence
already present against this – hardly a rational
approach.
There’s also possibly a cultural reason
that scientists have shied away from experimental investigations of
the inheritance of acquired characteristics. One of the most
notorious cases of scientific fraud is that of Paul Kammerer, who
worked in Austria in the first half of the 20th century. He claimed
that he had demonstrated the inheritance of acquired
characteristics in a species called the midwife toad.
Kammerer reported that when he changed
the conditions in which the toads bred, they developed ‘useful’
adaptations. These adaptations were structures on their forelimbs
called nuptial pads, which were black in colour. Unfortunately,
very few of the specimens were retained or stored well, and when a
rival scientist examined a specimen he found that India ink had
been injected into the pad. Kammerer denied all knowledge of the
contamination and killed himself shortly afterwards. This scandal
tainted an already controversial field1.
One of the statements in our potted
history of evolutionary theory was the following, ‘An acquired
change in phenotype would somehow have to ‘feed-back’ onto the DNA
script and change it really dramatically so that the acquired
characteristic could be transmitted in the space of just one
generation, from parent to child.’
It’s certainly hard to imagine how an
environmental influence on the cells of an individual could act at
a specific gene to change the base-pair sequence. But it’s all too
obvious that epigenetic modifications – be these DNA methylation or
alterations to the histone proteins – do indeed occur at specific
genes in response to the environmental influences on a cell. The
response to hormonal signalling that was mentioned in an earlier
chapter was an example of this. Typically, a hormone like oestrogen
will bind to a receptor on a cell from, for example, the breast.
The oestrogen and the receptor stay together and move into the
nucleus of the cell. They bind to specific motifs in DNA – A, C, G
and T bases in a particular sequence – which are found at the
promoters of certain genes. This helps to switch on the genes. When
it binds to these motifs, the oestrogen receptor also attracts
various epigenetic enzymes. These alter the histone modifications,
removing marks that repress gene expression and putting on marks
that tend to switch genes on. In this way, the environment, acting
via hormones, can change the epigenetic pattern at specific
genes.
These epigenetic modifications don’t
change the sequence of a gene, but they do alter how the gene is
expressed. This is, after all, the whole basis of developmental
programming for later disease. We know that epigenetic
modifications can be transmitted from a parent cell to a daughter
cell, as this is why there are no teeth in your eyeballs. If a
similar mechanism transmitted an environmentally-induced epigenetic
modification from an individual to their offspring, we would have a
mechanism for a sort of Lamarckian inheritance. An epigenetic (as
opposed to genetic) change would be passed down from parent to
child.
Heresy and the Dutch Hunger
Winter
It’s all very well to think about how
this could happen, but really we need to know if acquired
characteristics can actually be inherited in this way. Not
how does it happen, but the more basic question of
does it happen? Remarkably, there appear to be some specific
situations where this is indeed taking place. This doesn’t mean
that Darwinian/Mendelian models are wrong, it just means that, as
always, the world of biology is more complicated than we
imagined.
The scientific literature on this
contains some confusing terminology. Some early papers refer to
epigenetic transmission of an acquired trait but don’t seem to have
any evidence of DNA methylation changes, or histone alterations.
This isn’t sloppiness on the part of the authors. It’s because of
the different ways in which the word epigenetics has been used. In
the early papers the phrase ‘epigenetic transmission’ refers to
inheritance that cannot be explained by genetics. In these cases,
the word epigenetic is being used to describe the phenomenon, not
the molecular mechanism. To try to keep everything a little
clearer, we’ll use the phrase ‘transgenerational inheritance’ to
describe the phenomenon of transmission of an acquired
characteristic and only use ‘epigenetics’ to describe molecular
events.
Some of the strongest evidence for
transgenerational inheritance in humans comes from the survivors of
the Dutch Hunger Winter. Because the Netherlands has such excellent
medical infrastructure, and high standards of patient data
collection and retention, it has been possible for epidemiologists
to follow the survivors of the period of famine for many years.
Significantly, they were able to monitor not just the people who
had been alive in the Dutch Hunger Winter, but also their children
and their grandchildren.
This monitoring identified an
extraordinary effect. As we have already seen, when pregnant women
suffered malnutrition during the first three months of the
pregnancy, their babies were born with normal weight, but in
adulthood were at higher risk of obesity and other disorders.
Bizarrely, when women from this set of babies became mothers
themselves, their first born child tended to be heavier than in
control groups2,3. This is shown in Figure 6.1, where the relative sizes of the babies
have been exaggerated for clarity, and where we’ve given the women
arbitrary Dutch names.
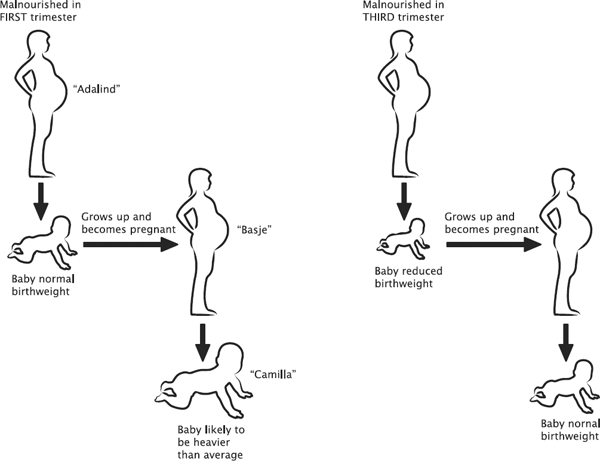
Figure 6.1 The
effects of malnutrition across two generations of children and
grandchildren of women who were pregnant during the Dutch Hunger
Winter. The timing of the malnutrition in pregnancy was critical
for the subsequent effects on body weight.
The effects on the birth weight of baby
Camilla shown at the bottom left are really odd. When Camilla was
developing, her mother Basje was presumably healthy. The only
period of malnutrition that Basje had suffered was twenty or more
years earlier, when she was going through her own first stages of
development in the womb. Yet it seems that this has an effect on
her own child, even though Camilla was never exposed to a period of
malnutrition during early development.
This seems like a good example of
transgenerational (Lamarckian) inheritance, but has it has been
caused by an epigenetic mechanism? Did an epigenetic change
(altered DNA methylation and/or variations in histone
modifications) that had occurred in Basje as a result of
malnutrition during her first twelve weeks of development in the
womb get passed on via the nucleus of her egg to her own child?
Maybe, but we shouldn’t ignore that there are other potential
explanations.
For example, there could be an
unidentified effect of the early malnutrition which means that when
pregnant, Basje will pass more nutrients than normal across the
placenta to her foetus. This would still create a transgenerational
effect – Camilla’s increased size – but it wouldn’t be caused by
Basje passing on an epigenetic modification to Camilla. It would be
caused by the conditions in the womb when Camilla was developing
and growing (the intrauterine environment).
It’s also important to remember that a
human egg is large. It contains a nucleus which is relatively small
in volume compared to the surrounding cytoplasm. Imagine a grape
inside a satsuma to gain some idea of relative sizes. The cytoplasm
carries out a lot of functions when an egg gets fertilised. Perhaps
something occurred during early developmental programming in Basje
that ultimately resulted in the cytoplasm of her eggs containing
something unusual. That might sound unlikely but egg production in
female mammals is actually initiated early in their own embryonic
development. The earliest stages of zygote development rely to a
large extent on the cytoplasm from the egg. An abnormality in the
cytoplasm could stimulate an unusual growth pattern in the foetus.
This again would result in transgenerational inheritance but not
through the direct transmission of an epigenetic
modification.
So we can see that there are various
mechanisms that could explain the inheritance patterns seen through
the maternal line in the Dutch Hunger Winter survivors. It would
help us to understand if epigenetics plays a role in acquired
inheritance if we could study a less complicated human situation.
Ideally, this would be a scenario where we don’t have to worry
about the effects of the intra-uterine environment, or the
cytoplasm of the egg.
Let’s hear it for fathers. Because men
don’t get pregnant, they can’t contribute to the developmental
environment of the foetus. Males also don’t contribute much
cytoplasm to the zygote. Sperm are very small and are almost all
nucleus – they look like little bullets with tails attached. So if
we see transgenerational inheritance from father to child, it isn’t
likely to be caused by intra-uterine or cytoplasmic effects. Under
these circumstances, an epigenetic mechanism would be an attractive
candidate for explaining transgenerational inheritance of an
acquired characteristic.
Greedy fellows in Sweden
Some data suggesting that male
transgenerational inheritance can occur in humans comes from
another historical study. There is a geographically isolated region
in Northern Sweden called Överkalix. In the late 19th and early
20th centuries there were periods of terrible food shortages
(caused by failed harvests, military actions and transport
inadequacies), interspersed with periods of great plenty.
Scientists have studied the mortality patterns for descendants of
people who were alive during these periods. In particular, they
analysed food intake during a stage in childhood known as the slow
growth period (SGP). All other factors being equal, children grow
slowest in the years leading up to puberty. This is a completely
normal phenomenon, seen in most populations.
Using historical records, the researchers
deduced that if food was scarce during a father’s SGP, his son was
at decreased risk of dying through cardiovascular disease (such as
stroke, high blood pressure or coronary artery disease). If, on the
other hand, a man had access to a surfeit of food during the SGP,
his grandsons were at increased risk of dying as a consequence of
diabetic illnesses4. Just like Camilla in the
Dutch Hunger Winter example, the sons and grandsons had an altered
phenotype (a change in the risk of death through cardiovascular
disease or diabetes) in response to an environmental challenge they
themselves had never experienced.
These data can’t be a result of the
intra-uterine environment nor of cytoplasmic effects, for the
reasons outlined earlier. Therefore, it seems reasonable to
hypothesise that the transgenerational consequences of food
availability in the grandparental generation were mediated via
epigenetics. These data are particularly striking when you consider
that the original nutritional effect happened when the boys were
pre-pubescent and so had not even begun to produce sperm. Even so,
they were able to pass an effect on to their sons and
grandsons.
However, there are some caveats around
this work on transgenerational inheritance through the male line.
In particular, there are risks involved in relying on old death
records, and extrapolating backwards through historical data.
Additionally, some of the effects that were observed were not
terribly large. This is frequently a problem when working with
human populations, along with all the other issues we have already
discussed, such as our genetic variability and the impossibility of
controlling environment in any major way. There is always the risk
that we draw inappropriate conclusions from our data, rather as we
believe Lamarck did with his studies on the families of
blacksmiths.
The heretical mouse
Is there an alternative way of
investigating transgenerational inheritance? If this phenomenon
also occurs in other species, it would give us a lot more
confidence that these effects are real. This is because experiments
in model systems can be designed to test specific hypotheses,
rather than just using the datasets that nature (or history)
provides.
This is where we come back to the
agouti mouse. Emma Whitelaw’s work showed that the variable
coat colour in the agouti mouse was due to an epigenetic
mechanism, specifically DNA methylation of a retrotransposon in the
agouti gene. Mice of different colour all had the same DNA
sequence, but a different degree of epigenetic modification at the
retrotransposon.
Professor Whitelaw decided to investigate
if the coat colour could be inherited. If it could, it would show
that it’s not only DNA that gets transmitted from parent to
offspring, but also epigenetic modifications to the genome. This
would provide a potential mechanism for the transgenerational
inheritance of acquired characteristics.
When Emma Whitelaw allowed female
agouti mice to breed, she found the effect that is shown in
Figure 6.2. For convenience, the picture
only shows the offspring who inherited the
Avy retrotransposon from their
mother, as this is the effect we are interested in.
If the mother had an unmethylated
Avy gene, and hence had yellow
fur, all her offspring also had either yellow fur, or slightly
mottled fur. She never had offspring who developed the very dark
fur associated with the methylation of the
retrotransposon.
By contrast, if the mother’s
Avy gene was heavily methylated,
resulting in her having dark fur, some of her offspring also had
dark fur. If both grandmother and mother had dark fur, then the
effect was even more pronounced. About a third of the final
offspring had dark fur, compared with the one in five shown in
Figure 6.2.
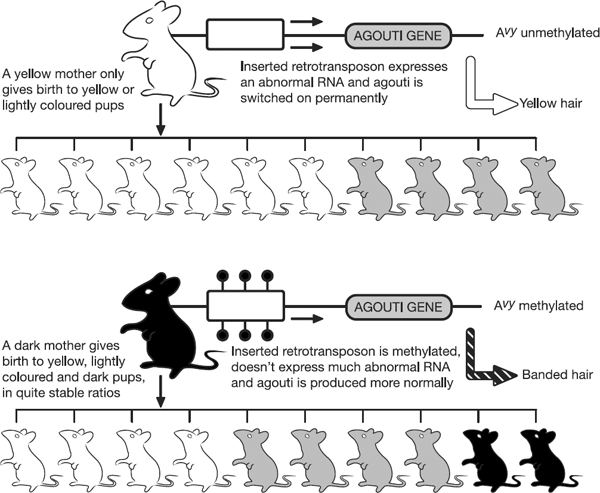
Figure 6.2 The coat
colour of genetically identical female mice influences the coat
colour of their offspring. Yellow female mice, in whom the agouti
gene is expressed continuously, due to low levels of DNA
methylation of the regulatory retrotransposon, never give birth to
dark pups. The epigenetically – rather than genetically –
determined characteristics of the mother influence her
offspring.
Because Emma Whitelaw was working on
inbred mice, she was able to perform this experiment multiple times
and generate hundreds of genetically identical offspring. This was
important, as the more data points we have in an experiment, the
more we can rely on the findings. Statistical tests showed that the
phenotypic differences between the genetically identical groups
were highly significant. In other words, it was very unlikely that
the effects occurred by chance5.
The results from these experiments showed
that an epigenetically-mediated effect (the DNA
methylation-dependent coat pattern) in an animal was transmitted to
its offspring. But did the mice actually inherit directly an
epigenetic modification from their mother?
There was a possibility that the effects
seen were not directly caused by inheritance of the epigenetic
modification at the Avy
retrotransposon, but through some other mechanism. When the
agouti gene is switched on too much, it doesn’t just cause
yellow fur. Agouti also mis-regulates the expression of
other genes, which ultimately results in the yellow mice being fat
and diabetic. So it’s likely that the intra-uterine environment
would be different between yellow and dark pregnant females, with
different nutrient availability for their embryos. The nutrient
availability could itself change how particular epigenetic marks
get deposited at the Avy
retrotransposon in the offspring. This would look like epigenetic
inheritance, but actually the pups wouldn’t have directly inherited
the DNA methylation pattern from their mother. Instead, they’d just
have gone through a similar developmental programming process in
response to nutrient availability in the uterus.
Indeed, at the time of Emma Whitelaw’s
work, scientists already knew that diet could influence coat colour
in agouti mice. When pregnant agouti mice are fed a
diet rich in the chemicals that can supply methyl groups to the
cells (methyl donors), the ratios of the differently coloured pups
changes6. This is presumably because
the cells are able to use more methyl groups, and deposit more
methylation on their DNA, hence shutting down the abnormal
expression of agouti. This meant that the Whitelaw group had
to be really careful to control for the effect of intrauterine
nutrition in their experiments.
In one of those experiments that simply
aren’t possible in humans, they transferred fertilised eggs
obtained from yellow mothers and implanted them into dark females,
and vice versa. In every case, the distribution of coat patterns in
the offspring was the same as was to be expected from the egg
donor, i.e. the biological mother, rather than the surrogate. This
showed unequivocally that it wasn’t the intra-uterine environment
that controlled the coat patterning. By using complex breeding
schemes, they also demonstrated that the inheritance of the coat
pattern was not due to the cytoplasm in the egg. Taken together,
the most straightforward interpretation of these data is that
epigenetic inheritance has taken place. In other words, an
epigenetic modification (probably DNA methylation) was transferred
along with the genetic code.
This transfer of the phenotype from one
generation to the next wasn’t perfect – not all the offspring
looked exactly the same as their mother. This implies that the DNA
methylation that controls the expression of the agouti
phenotype wasn’t entirely stable down the generations. This is
quite analogous to the effects we see in suspected cases of human
transgenerational inheritance, such as the Dutch Hunger Winter. If
we look at a large enough number of people in our study group we
can detect differences in birth weight between various groups, but
we can’t make absolute predictions about a single
individual.
There is also an unusual gender-specific
phenomenon in the agouti strain. Although coat pattern
showed a clear transgenerational effect when it was passed on from
mother to pup, no such effect was seen when a male mouse passed on
the Avy retrotransposon to his
offspring. It didn’t matter if a male mouse was yellow, lightly
mottled or dark. When he fathered a litter, there were likely to be
all the different patterns of colour in his offspring.
But there are other examples of
epigenetic inheritance transmitted from both males and females. The
kinked tail phenotype in mice, which is caused by variable
methylation of a retrotransposon in the
AxinFu (Axin fused) gene, can be
transmitted by either the mother or the father7. This
makes it unlikely that transgenerational inheritance of this
characteristic is due to intra-uterine or cytoplasmic influences,
because fathers don’t really contribute much to these. It’s far
more likely that there is the transmission of an epigenetic
modification at the AxinFu gene
from either parent to offspring.
These model systems have been really
useful in demonstrating that transgenerational inheritance of a
non-genetic phenotype does actually occur, and that this takes
place via epigenetic modifications. This is truly revolutionary. It
confirms that for some very specific situations Lamarckian
inheritance is taking place, and we have a handle on the molecular
mechanism behind it. But the agouti and kinked tail
phenotypes in mice both rely on the presence of specific
retrotransposons in the genome. Are these special cases, or is
there a more general effect in play? Once again, we return to
something that has a bit more immediate relevance for us all.
Food.
The epigenetics of obesity
As we all know, an obesity epidemic is
developing. It’s spreading worldwide, although it’s advancing at a
particularly fast rate in the more industrialised societies. The
frankly terrifying graph in Figure 6.3
displays the UK figures for 20078, showing that about two out
of every three adults is overweight (body mass index of 25 or over)
or obese (body mass index of 30 or over). The situation is even
worse in the USA. Obesity is associated with a wide range of health
problems including cardiovascular disease and type 2 diabetes.
Obese individuals over the age of 40 will die, on average, 6 to 7
years earlier than non-obese people9.
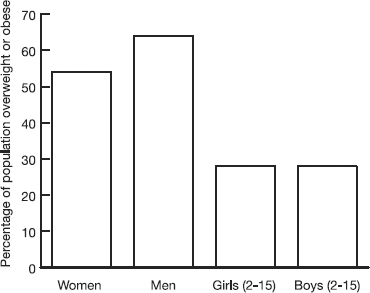
Figure 6.3 The
percentage of the UK population that was overweight or obese in
2007.
The data from the Dutch Hunger Winter and
other famines support the idea that poor nutrition during pregnancy
has effects on offspring, and that these consequences can be
transmitted to subsequent generations as well. In other words, poor
nutrition can have epigenetic effects on later generations. The
data from the Överkalix cohort, although more difficult to
interpret, suggested that excess consumption at key points in a
boy’s life can have adverse consequences for later generations. Is
it possible that the obesity epidemic in the human population will
have knock-on effects for children and grandchildren? As we don’t
really want to wait 40 years to work this out, scientists are again
turning to animal models to try to gain some useful
insights.
The first animal data suggested that
nutrition might not have much effect transgenerationally. The
change in coat pattern of pups when pregnant agouti mice
were given diets high in methyl donors didn’t transmit to the next
generation10. But perhaps this is too
specialised a model. In 2010, two papers were published that should
at least give us pause for thought. They were published in two of
the best journals in the world – Nature and Cell. In
both cases, the researchers overfed male animals and then monitored
the effects on their offspring. By restricting their experiments to
males, they didn’t need to worry about the intra-uterine and
cytoplasmic complications that cause such (metaphorical) headaches
if studying females.
One of the studies used a breed of rat
called Sprague-Dawley. This is an albino rat, with a chilled-out
temperament that makes it easy to keep and handle. In the
experiments male Sprague-Dawleys were given a high-fat diet, and
allowed to mate with females who had been fed an ordinary diet. The
over-fed males were overweight (hardly a surprise), had a high
percentage of fat to muscle and had many of the symptoms found in
type 2 diabetes in humans. Offspring were normal weight but they
too had the diabetes-type abnormalities11.
Many of the genes that control metabolism and how mammals burn fuel
were mis-regulated in these offspring. For reasons that aren’t
understood, it was particularly the daughters that showed this
effect.
A completely independent group studied
the effects of diet in an inbred mouse strain. Male mice were fed a
diet that was abnormally low in protein. The diet had an increased
percentage of sugar to make up for this. The males were mated to
females on a normal diet. The researchers examined the expression
of genes in the liver (the body’s major organ when it comes to
metabolism) in three-week-old pups from these matings. Analysing
large numbers of mouse pups, they found that the regulation of many
of the genes involved in metabolism was abnormal in the offspring
of the males that had been fed the modified diet12.
They also found changes in the epigenetic modifications in the
livers of these pups.
So, both these studies show us that, at
least in rodents, a father’s diet can directly influence the
epigenetic modifications, gene expression and health of his
offspring. And not because of environment – this isn’t like the
human example of a child getting fat because their Dad only ever
feeds them super-sized portions of burgers and chips. It’s a direct
effect and it occurred so frequently in the rats and mice that it
can’t have been due to diet-induced mutations, they just don’t
happen at that sort of rate. So the most likely explanation is that
diet induces epigenetic effects that can be transmitted from father
to child. Although the data are quite preliminary, the results from
the mouse study in particular support this.
If you look at all the data in its
entirety – from humans to rodents, from famine to feast – a quite
worrying pattern emerges. Maybe the old saw of ‘we are what we eat’
doesn’t go far enough. Maybe we’re also what our parents ate and
what their parents ate before them.
This might make us wonder if there is any
point following advice on healthy living. If we are all victims of
epigenetic determinism, this would suggest that our dice have
already been rolled, and we are just at the mercy of our ancestors’
methylation patterns. But this is far too simplistic a model.
Overwhelming amounts of data show that the health advice issued by
government agencies and charities – eating a healthy diet rich in
fruit and vegetables, getting off the sofa, not smoking – is
completely sound. We are complex organisms, and our health and life
expectancy are influenced by our genome, our epigenome and our
environment. But remember that even in the inbred agouti
mice, kept under standardised conditions, researchers couldn’t
predict exactly how yellow or how fat an individual mouse in a
newborn litter would become. Why not do everything that we can to
improve our chances of a healthy and long life? And if we are
planning to have children, don’t we want to do whatever we can to
nudge them that bit closer to good health?
There will always be things we can’t
control, of course. One of the best-documented examples of an
environmental factor that has epigenetic consequences, lasting at
least four generations, is an environmental toxin. Vinclozolin is a
fungicide, which tends to be used particularly frequently in the
wine industry. If it gets into mammals it is converted into a
compound that binds to the androgen receptor. This is the receptor
that binds testosterone, the male hormone that is vital for sexual
development, sperm production and a host of other effects in males.
When vinclozolin binds to the androgen receptor, it prevents
testosterone from transmitting its usual signals to the cells, and
so blocks the normal effects of the hormone.
If vinclozolin is given to pregnant rats
at the time when the testes are developing in the embryos, the male
offspring are born with testicular defects and have reduced
fertility. The same effect is found for the next three
generations13. About 90 per cent of the
male rats are affected, which is far too high a percentage to be
caused by classic DNA mutation. Even the highest known rates of
mutation, at particularly sensitive regions of the genome, are at
least ten-fold less frequent than this. In these rat experiments,
only one generation was exposed to vinclozolin, yet the effect
lasted for at least four generations, so this is another example of
Lamarckian inheritance. Given the male transmission pattern, it is
likely this is another example of an epigenetic inheritance
mechanism. A follow-on publication from the same research group has
identified regions of the genome where vinclozolin treatment leads
to unusual DNA methylation patterns14.
The rats in the studies described above
were treated with high doses of vinclozolin. These were much larger
than humans are believed to encounter in the environment.
Nonetheless, effects such as these are one of the reasons why some
authorities are beginning to investigate if artificial hormones and
hormone disrupters in the environment (from excretion of chemicals
present in the contraceptive pill, to certain pesticides) have the
potential to cause subtle, but potentially transgenerational
effects in the human population.